Optimization of the surface code design for Majorana-based qubits
1University of Southern California, Los Angeles, CA, USA
2Microsoft Quantum and Microsoft Research, Redmond, WA, USA
Published: | 2020-10-28, volume 4, page 352 |
Eprint: | arXiv:2007.00307v2 |
Doi: | https://doi.org/10.22331/q-2020-10-28-352 |
Citation: | Quantum 4, 352 (2020). |
Find this paper interesting or want to discuss? Scite or leave a comment on SciRate.
Abstract
The surface code is a prominent topological error-correcting code exhibiting high fault-tolerance accuracy thresholds. Conventional schemes for error correction with the surface code place qubits on a planar grid and assume native CNOT gates between the data qubits with nearest-neighbor ancilla qubits.
Here, we present surface code error-correction schemes using $\textit{only}$ Pauli measurements on single qubits and on pairs of nearest-neighbor qubits. In particular, we provide several qubit layouts that offer favorable trade-offs between qubit overhead, circuit depth and connectivity degree. We also develop minimized measurement sequences for syndrome extraction, enabling reduced logical error rates and improved fault-tolerance thresholds.
Our work applies to topologically protected qubits realized with Majorana zero modes and to similar systems in which multi-qubit Pauli measurements rather than CNOT gates are the native operations.
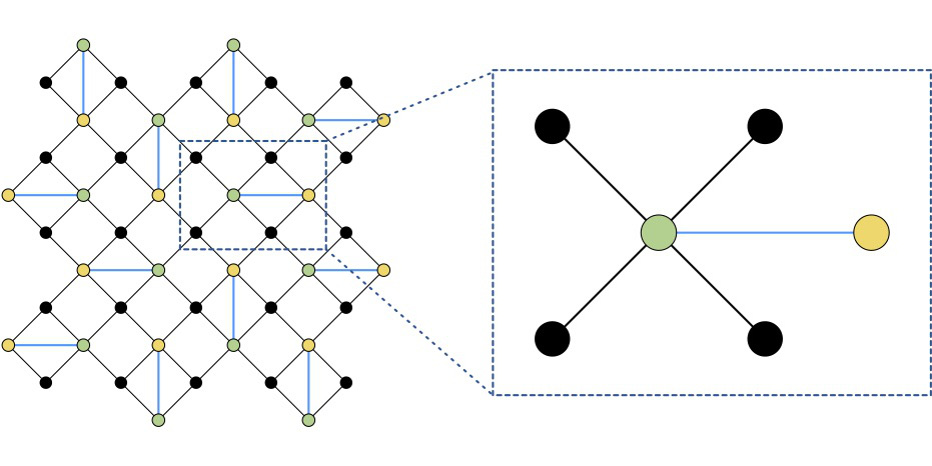
Featured image: The windmill layout for implementing the distance-5 surface code with measurement-based qubits. Black nodes are data qubits; at the center of each X-type or Z-type plaquette, there is a green or yellow ancilla qubit, respectively. The measurement of a plaquette will require two-qubit measurements between the data qubits and the center ancilla (black edges), and between the center ancilla and its neighbor ancilla (blue edge).
► BibTeX data
► References
[1] A. Y. Kitaev, Ann. Phys. 303, 2 (2003), quant-ph/9707021.
https://doi.org/10.1016/S0003-4916(02)00018-0
arXiv:quant-ph/9707021
[2] E. Dennis, A. Kitaev, A. Landahl, and J. Preskill, J. Math. Phys. 43, 4452 (2002), quant-ph/0110143.
https://doi.org/10.1063/1.1499754
arXiv:quant-ph/0110143
[3] A. G. Fowler, M. Mariantoni, J. M. Martinis, and A. N. Cleland, Phys. Rev. A 86, 032324 (2012), 1208.0928.
https://doi.org/10.1103/PhysRevA.86.032324
arXiv:1208.0928
[4] R. Raussendorf and J. Harrington, Phys. Rev. Lett. 98, 190504 (2007), quant-ph/0610082.
https://doi.org/10.1103/PhysRevLett.98.190504
arXiv:quant-ph/0610082
[5] A. G. Fowler, A. M. Stephens, and P. Groszkowski, Phys. Rev. A 80, 052312 (2009), 0803.0272.
https://doi.org/10.1103/PhysRevA.80.052312
arXiv:0803.0272
[6] N. Delfosse and G. Zémor, Physical Review Research 2, 033042 (2020), 1703.01517.
https://doi.org/10.1103/PhysRevResearch.2.033042
arXiv:1703.01517
[7] N. Delfosse and N. H. Nickerson, 2017, 1709.06218.
arXiv:1709.06218
[8] T. Karzig, C. Knapp, R. M. Lutchyn, P. Bonderson, M. B. Hastings, C. Nayak, J. Alicea, K. Flensberg, S. Plugge, Y. Oreg, C. M. Marcus, and M. H. Freedman, Phys. Rev. B 95, 235305 (2017), 1610.05289.
https://doi.org/10.1103/PhysRevB.95.235305
arXiv:1610.05289
[9] C. Knapp, M. Beverland, D. I. Pikulin, and T. Karzig, Quantum 2, 88 (2018), 1806.01275.
https://doi.org/10.22331/q-2018-09-03-88
arXiv:1806.01275
[10] Y. Li, Physical Review Letters 117, 120403 (2016), 1512.05089.
https://doi.org/10.1103/PhysRevLett.117.120403
arXiv:1512.05089
[11] S. Plugge, L. Landau, E. Sela, A. Altland, K. Flensberg, and R. Egger, Phys. Rev. B 94, 174514 (2016), 1606.08408.
https://doi.org/10.1103/PhysRevB.94.174514
arXiv:1606.08408
[12] D. Litinski, M. S. Kesselring, J. Eisert, and F. von Oppen, Phys. Rev. X 7, 031048 (2017), 1704.01589.
https://doi.org/10.1103/PhysRevX.7.031048
arXiv:1704.01589
[13] A. G. Fowler, D. S. Wang, and L. C. L. Hollenberg, Quant. Info. Comput. 11, 8 (2011), 1004.0255.
https://doi.org/10.26421/QIC11.1-2
arXiv:1004.0255
[14] M. Newman, L. A. de Castro, and K. R. Brown, Quantum 4, 295 (2020), 1909.11817.
https://doi.org/10.22331/q-2020-07-13-295
arXiv:1909.11817
[15] A. G. Fowler, 2013, 1310.0863.
arXiv:1310.0863
[16] N. Delfosse and J.-P. Tillich, in 2014 IEEE Int. Symp. Info. (IEEE, 2014) pp. 1071–1075, 1401.6975.
https://doi.org/10.1109/ISIT.2014.6874997
arXiv:1401.6975
[17] S. Huang and K. R. Brown, Phys. Rev. A 101, 042312 (2020), 1911.11317.
https://doi.org/10.1103/PhysRevA.101.042312
arXiv:1911.11317
[18] S. Huang, M. Newman, and K. R. Brown, Phys. Rev. A 102, 012419 (2020), 2004.04693.
https://doi.org/10.1103/PhysRevA.102.012419
arXiv:2004.04693
[19] J. MacWilliams, Bell Syst. Tech. J. 42, 79 (1963).
https://doi.org/10.1002/j.1538-7305.1963.tb04003.x
[20] S. Bravyi and A. Vargo, Phys. Rev. A 88, 062308 (2013), 1308.6270.
https://doi.org/10.1103/PhysRevA.88.062308
arXiv:1308.6270
Cited by
[1] Craig Gidney, Michael Newman, Austin Fowler, and Michael Broughton, "A Fault-Tolerant Honeycomb Memory", Quantum 5, 605 (2021).
[2] Oscar Higgott, Thomas C. Bohdanowicz, Aleksander Kubica, Steven T. Flammia, and Earl T. Campbell, "Improved Decoding of Circuit Noise and Fragile Boundaries of Tailored Surface Codes", Physical Review X 13 3, 031007 (2023).
[3] Craig Gidney, "Stim: a fast stabilizer circuit simulator", Quantum 5, 497 (2021).
[4] Qian Xu, Guo Zheng, Yu-Xin Wang, Peter Zoller, Aashish A. Clerk, and Liang Jiang, "Autonomous quantum error correction and fault-tolerant quantum computation with squeezed cat qubits", npj Quantum Information 9 1, 78 (2023).
[5] Kaavya Sahay, Jahan Claes, and Shruti Puri, "Tailoring Fusion-Based Error Correction for High Thresholds to Biased Fusion Failures", Physical Review Letters 131 12, 120604 (2023).
[6] Craig Gidney, "A Pair Measurement Surface Code on Pentagons", Quantum 7, 1156 (2023).
[7] Jeongwan Haah and Matthew B. Hastings, "Boundaries for the Honeycomb Code", Quantum 6, 693 (2022).
[8] Aleksander Kubica and Michael Vasmer, "Single-shot quantum error correction with the three-dimensional subsystem toric code", Nature Communications 13 1, 6272 (2022).
[9] Theerapat Tansuwannont and Debbie Leung, "Achieving Fault Tolerance on Capped Color Codes with Few Ancillas", PRX Quantum 3 3, 030322 (2022).
[10] Qian Xu, Nam Mannucci, Alireza Seif, Aleksander Kubica, Steven T. Flammia, and Liang Jiang, "Tailored XZZX codes for biased noise", Physical Review Research 5 1, 013035 (2023).
[11] Xiu-Bo Chen, Li-Yun Zhao, Gang Xu, Xing-Bo Pan, Si-Yi Chen, Zhen-Wen Cheng, and Yi-Xian Yang, "Low-overhead fault-tolerant error correction scheme based on quantum stabilizer codes", Chinese Physics B 31 4, 040305 (2022).
[12] Edward H. Chen, Theodore J. Yoder, Youngseok Kim, Neereja Sundaresan, Srikanth Srinivasan, Muyuan Li, Antonio D. Córcoles, Andrew W. Cross, and Maika Takita, "Calibrated Decoders for Experimental Quantum Error Correction", Physical Review Letters 128 11, 110504 (2022).
[13] Craig Gidney, Michael Newman, and Matt McEwen, "Benchmarking the Planar Honeycomb Code", Quantum 6, 813 (2022).
[14] Kaavya Sahay and Benjamin J. Brown, "Decoder for the Triangular Color Code by Matching on a Möbius Strip", PRX Quantum 3 1, 010310 (2022).
[15] Michael E. Beverland, Aleksander Kubica, and Krysta M. Svore, "Cost of Universality: A Comparative Study of the Overhead of State Distillation and Code Switching with Color Codes", PRX Quantum 2 2, 020341 (2021).
[16] Adam Paetznick, Christina Knapp, Nicolas Delfosse, Bela Bauer, Jeongwan Haah, Matthew B. Hastings, and Marcus P. da Silva, "Performance of Planar Floquet Codes with Majorana-Based Qubits", PRX Quantum 4 1, 010310 (2023).
[17] Dongxiao Quan, Chensong Liu, Xiaojie Lv, and Changxing Pei, "Implementation of Fault-Tolerant Encoding Circuit Based on Stabilizer Implementation and “Flag” Bits in Steane Code", Entropy 24 8, 1107 (2022).
[18] Michael Beverland, Vadym Kliuchnikov, and Eddie Schoute, "Surface Code Compilation via Edge-Disjoint Paths", PRX Quantum 3 2, 020342 (2022).
[19] Linnea Grans-Samuelsson, Ryan V. Mishmash, David Aasen, Christina Knapp, Bela Bauer, Brad Lackey, Marcus P. da Silva, and Parsa Bonderson, "Improved Pairwise Measurement-Based Surface Code", arXiv:2310.12981, (2023).
[20] David Aasen, Jeongwan Haah, Zhi Li, and Roger S. K. Mong, "Measurement Quantum Cellular Automata and Anomalies in Floquet Codes", arXiv:2304.01277, (2023).
The above citations are from Crossref's cited-by service (last updated successfully 2024-07-03 03:25:10) and SAO/NASA ADS (last updated successfully 2024-07-03 03:25:11). The list may be incomplete as not all publishers provide suitable and complete citation data.
This Paper is published in Quantum under the Creative Commons Attribution 4.0 International (CC BY 4.0) license. Copyright remains with the original copyright holders such as the authors or their institutions.